Quantum Systems
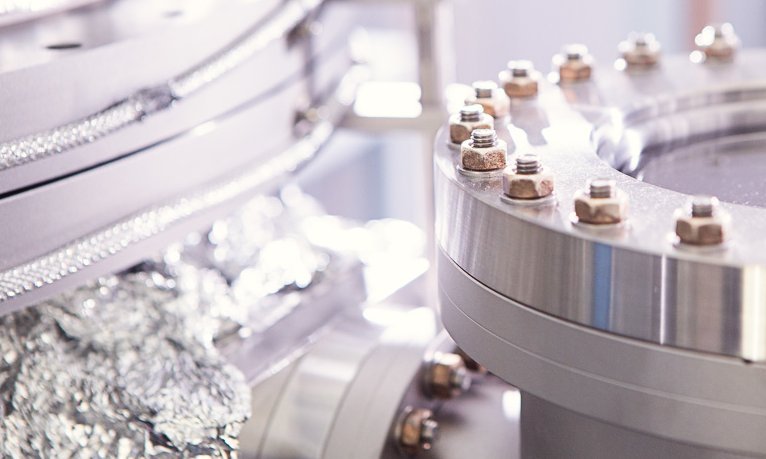
Today we are witnessing a scientific and technological revolution, which has been enabled by the realization and manipulation of engineered quantum systems and their use in quantum information processing, communication and sensing. This development has led to the novel research field of Quantum Science and Technology (QST), which advances the understanding of fundamental principles of quantum systems and explores their practical applications.
Quantum systems based on engineered solid-state systems and circuits have attracted enormous interest because they provide one of the most promising hardware platforms for quantum computers, quantum communication systems, and applications in quantum sensing. We study the fundamental properties of various solid-state systems based on superconducting circuits, spin systems, nano-mechanical systems as well as hybrid systems thereof. We also use them for the realization of quantum bits and circuits. Key topics addressed by our research are decoherence mechanisms, the realization of strong and ultra-strong coupling between quantized solid-state excitations and microwave photons (e.g. magnon-photon, magnon-phonon, phonon-photon coupling), as well as the optimization of the manipulation, control and readout of solid-state quantum bits. Highlights of our research have been the first demonstration of ultra-strong coupling in superconducting circuit QED, the first demonstration of strong magnon-photon coupling, or the observation of echo trains in pulsed electron spin resonance of a strongly coupled spin ensemble.
For key quantum technology platforms (e.g. superconducting circuits, NV centers, quantum dots), microwaves intrinsically allow for zero frequency conversion loss, since they are the natural frequency scale. Therefore, our research aims at developing novel components, experimental techniques, and theory models building on the quantum properties of continuous-variable propagating microwaves. Our long-term visions include distributed quantum computing & communication via microwave quantum local area networks (QLANs) as well as sensing applications based on the illumination of an object with quantum microwaves (quantum radar). To this end, we already developed the dual path method for state tomography of propagating quantum microwaves and demonstrated path entanglement, one- and two-mode squeezing, the displacement operation or the implementation of the remote state preparation protocol.
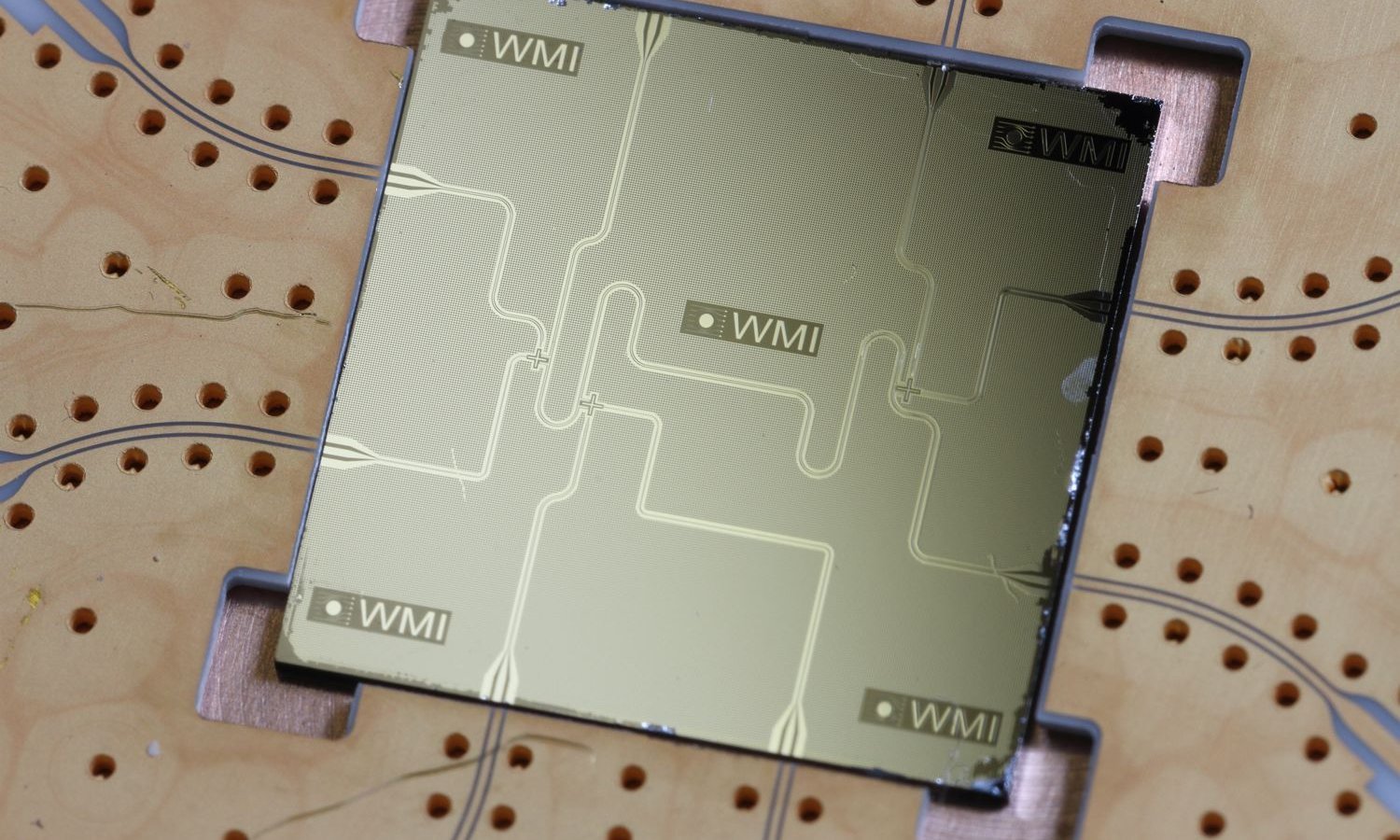
We study the foundations and applications of superconducting quantum circuits. The latter include the astonishing demonstration of textbook quantum mechanics as well as quantum information processing (QIP) and quantum simulation. Our research does not only address the foundations of quantum information systems and superconducting quantum technology, but also key fundamental questions regarding quantum coherence, quantum dynamics, and decoherence mechanisms in solid state quantum systems. Furthermore, it requires extremely sensitive measurements at millikelvin temperatures.
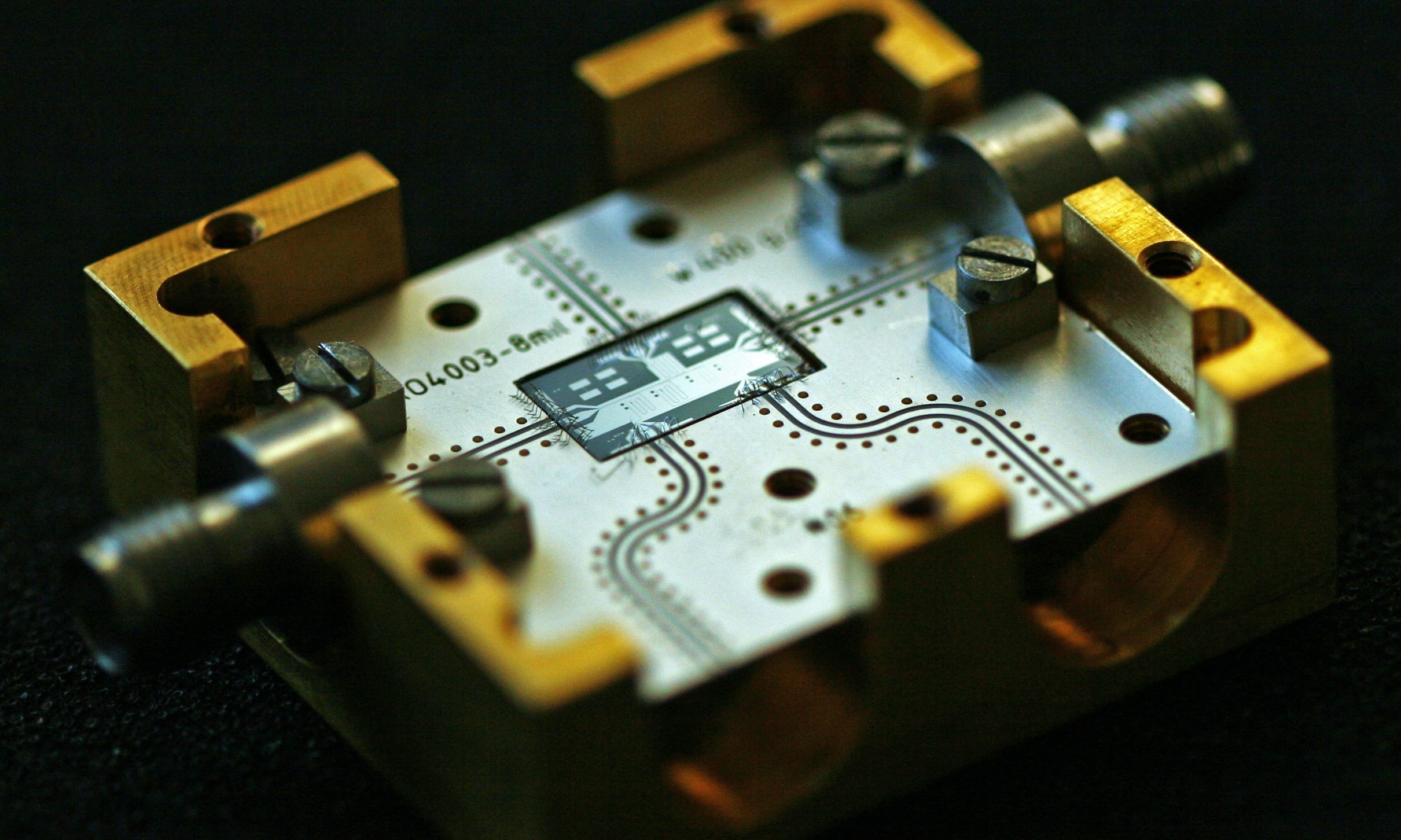
At the Walther-Meissner-Institute, we explore two coupling stategies for the realisation of the nano-electromechanical interaction:
- a capacitive coupling scheme, where the displacement of the nano-string resonator changes the overall capacitance of the superconducting electronic circuit, and
- an inductive coupling scheme, where we utilize the tunable inductance of a superconducting interference device to realize this purpose.
With these integrated nano-electromechanical devices, we have demonstrated force sensitivities down to aN/sqrt(Hz) and coupling rates in the tens of kHz range. We utilize these platforms to investigate and understand the interaction itself, study the mechanical properties of the materials involved and realize literal quantum mechanical states.
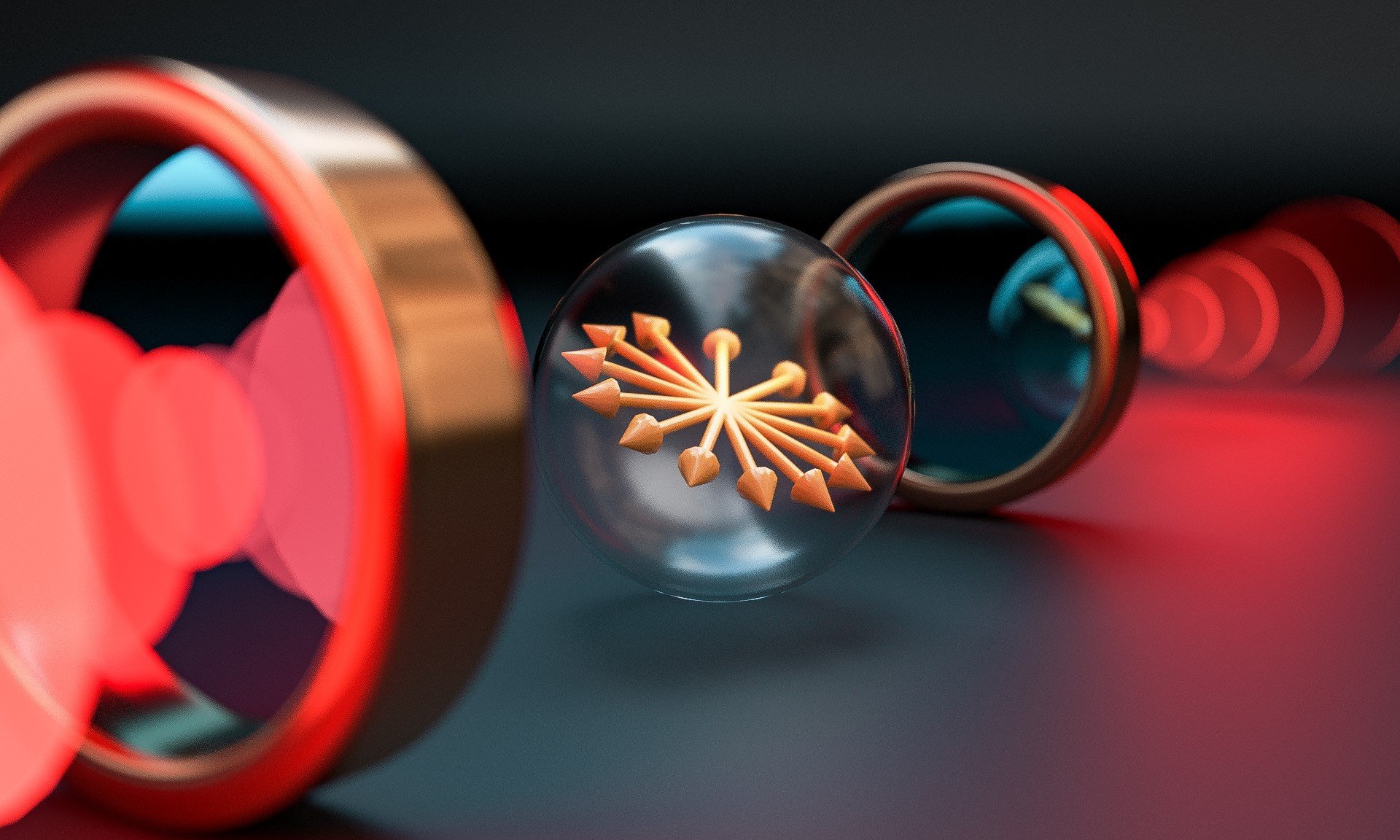
At the Walther-Meissner-Institute we pioneered research in the direction of strong magnon-photon interaction, which is key to investigate magnons on the quantum level, but also gives deep insight in the hybridization of magonic and phonic states, an aspect which we have intensely researched by combining this hybrid system with electical readout techniques.
Spin ensembles based on paramagnetic centers are complementary to their exchange coupled counterpart. Although, the coupling is less intense, they still can be operated in the strong coupling regime. Due to their extreme coherence times, these spin systems are discussed for quantum memory or quantum transduction applications.
In addition, we study magnon-phonon hybrids based on acoustic resonators and magnetic thin films, as well as coupled nano-string resonator networks.
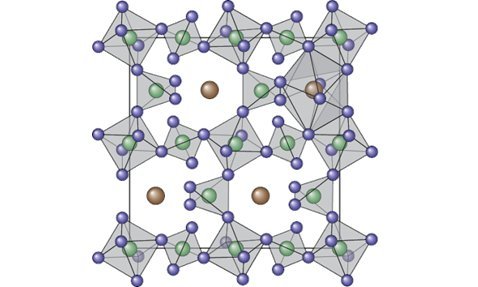
Like magnetism and superconductivity, those quantum phases are not only of high interest from the fundamental science perspective, but also have potential applications in quantum science and technology. We fabricate thin film and multilayer quantum materials, including magnetically ordered insulators (e.g. Y3Fe5O12 or α-Fe2O3), spin-orbit driven materials (e.g. Sr2IrO4), or Dzyaloshinskii-Moriya-active interfaces...