Projects
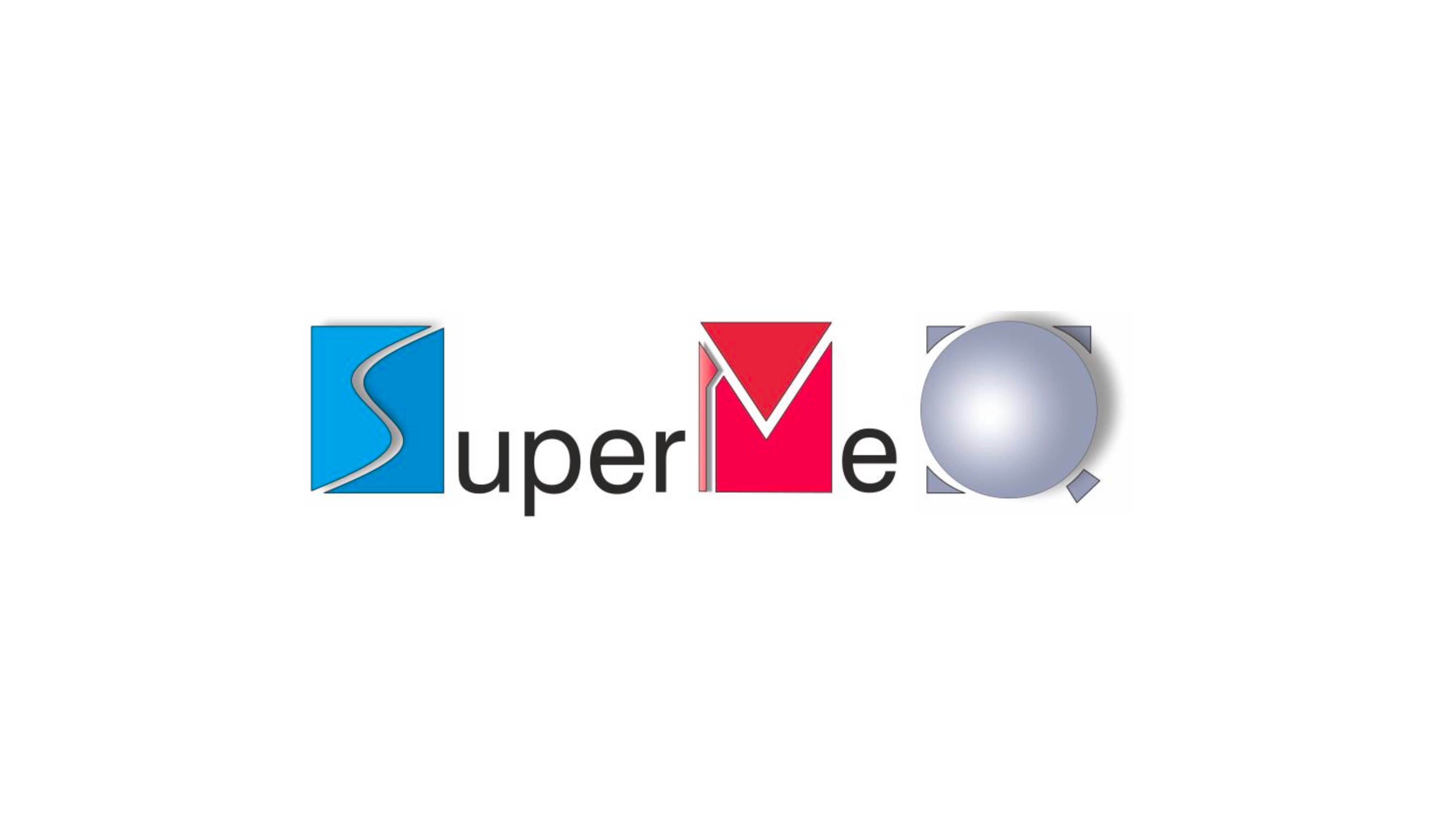
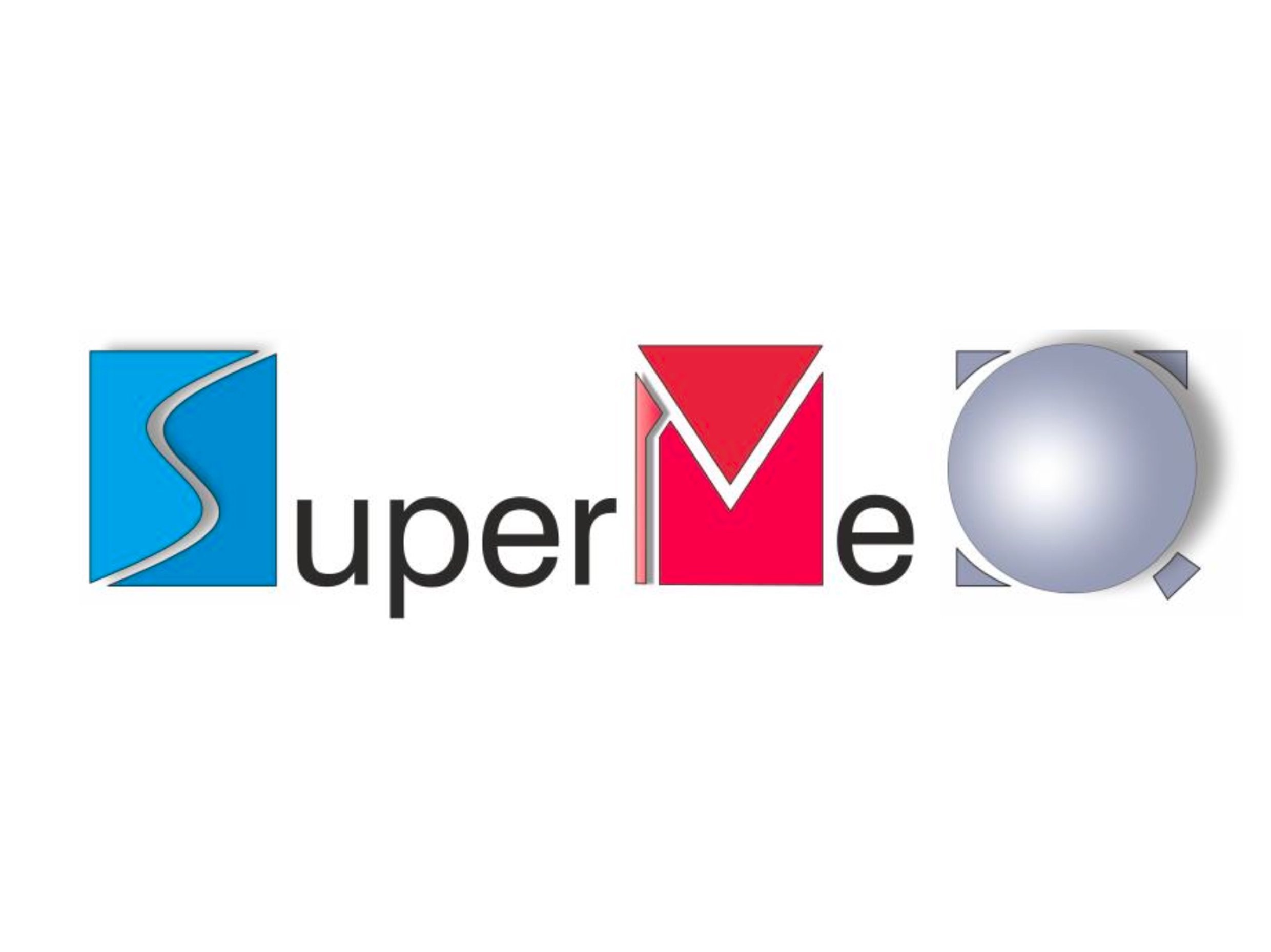
Within the EU project SuperMeQ, WMI collaborates with partners from Chalmers University of Technology, the University of Vienna, the University of Innsbruck, the Austrian Academy of Sciences, the Karlsruhe Institute of Technology, and the Universitat Autonòma de Barcelona as well as the industry partner Infineon. Our project follows a unique approach by realizing two complementary experimental platforms that are tailored to our goals and that are mutually beneficial through parallel development: (a) magnetically levitated superconducting microparticles that access a mass regime spanning more than seven orders of magnitude between picogram and sub-milligram masses, and that are expected to exhibit ultra-low mechanical decoherence, and (b) integrated clamped magnetic or superconducting mechanical resonators that are expected to reach strong vacuum coupling rates, two orders of magnitude larger than the state-of-the-art. Key in each of these approaches is that we will couple both types of mechanical resonator inductively to superconducting quantum circuits, which allow for full quantum control over the center-of-mass degree of freedom of the mechanical resonators. Our project results will lead to a breakthrough in the development and growth of novel quantum sensing technologies and give new insights into foundational aspects of quantum physics.
The WMI contributes to this project the following areas: (i) pushing optomechanics to the strong single photon coupling regime, (ii) the development of superconducting quantum circuits for the readout of massive objects, and (iii) the generation of non-classical states for sensing. The established nano-electromechanical systems based on nano-strings developed within the WMI in combination with the inductive readout technique developed at WMI provide an exquisite starting position for the successful realization of the goals of SuperMEQ. In addition, the long-standing and established know-how of the institute in areas such as low-temperature technology and vibration isolation techniques contribute further to those key challenges.
Coordinator: Witlef Wieczorek (Chalmers University)
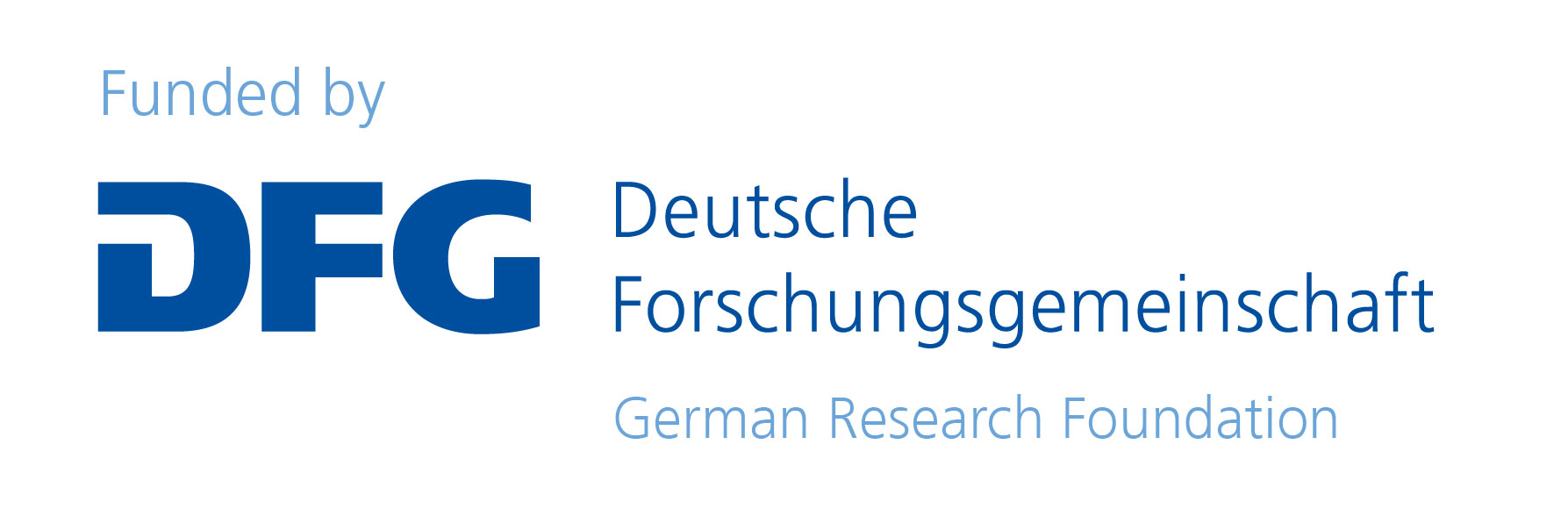
The research center started in October 2023 and brings together researchers at the University of Augsburg, the Technical University of Munich, the Max Planck Institute for Solid State Physics, the University of Leipzig, the University of Tokyo, and the Walther-Meissner-Institute of the Bavarian Academy of Sciences and Humanities. In 18 projects, 32 PhD and postdoctoral researchers will address questions on magnetic band topology, entangled states of matter, and non-equilibrium dynamics in selected solid-state systems. The basic guiding principle is that a reduction of the degrees of freedom through suitable constraints can yield exciting physical phenomena. Our approach will facilitate the realization as well as detection of quantum entanglement and the exploration of novel quantum effects in solid-state materials with the long-term goal of stabilizing them at practical conditions, thus fostering applications on a broad scale.
At the Walther-Meissner-Institute, a team around Hans Huebl and Stephan Geprägs will team up with Christian Pfleiderer and Mark Wilde from the School of Natural Sciences of the Technical University of Munich to investigate the properties of quantum materials in non-equilibrium situations using multiple excitation and detection techniques. For example, we plan to drive low-energy excitations by intense microwave radiation while the response function is probed with a second probe. This approach aims at (i) investigating and understanding the coupling between spin, orbital, lattice or nuclear spin degrees of freedom under intense resonant microwave radiation, (ii) a targeted driving of quantum excitations such as topological magnons, orbitons or topological electronic quasiparticles, (iii) inducing non-thermal melting of long-range order by intense microwave radiation, and (iv) realization of dynamical forms of quantum order in bulk materials such as time crystals or many-body localization.
Coordinator: Prof. Dr. István Kézsmárki (University of Augsburg)
Our first direction is based on superconducting cavity-qubits systems. We made progress with modelling and fabricating superconducting aluminum cavities coupled to niobium-aluminum transmon qubits, which are known for their long coherence times and reproducibility. In this context, we also focus on preliminary theory simulations of relevant remote entanglement protocols, which are based on the illumination of remote qubits with propagating two-mode squeezed microwave signals.
Our second direction is focused on transduction between microwave and optical frequency domains while trying to preserve fragile quantum correlations. Here, we would like to exploit magneto-electro-mechanical nanodevices. These devices rely on a hybrid frequency conversion by combining opto-mechanics and opto-magnonics. This conversion concept uses a combined three-step conversion process, involving the coupling of microwave photons with magnons, the coupling of the magnonic and tailored phononic degrees of freedom harnessing magneto-elasticity, and the opto-mechanical interaction linking the photonic and phononic degree of freedom. In particular, the three-step conversion should allow us to overcome the cooperativity matching criterion of a one-step conversion process and, hereby, will allow for much larger bandwidths. Along these lines we are also collaborating with the group of Prof. Jonathan Finley towards microwave-to-optical transduction schemes based on quantum dot molecules in semiconductor heterostructures. Coupling quantum dot molecules to microwave photons in superconducting waveguide resonators via the ac-Stark effect leads to sidebands in the optical spectrum, which can be used for coherent transduction.
In summary, we are currently in the process of developing two complementary approaches towards distributing quantum correlations over long distances with a final aim to form hybrid, microwave-microwave and microwave-optical, quantum networks.
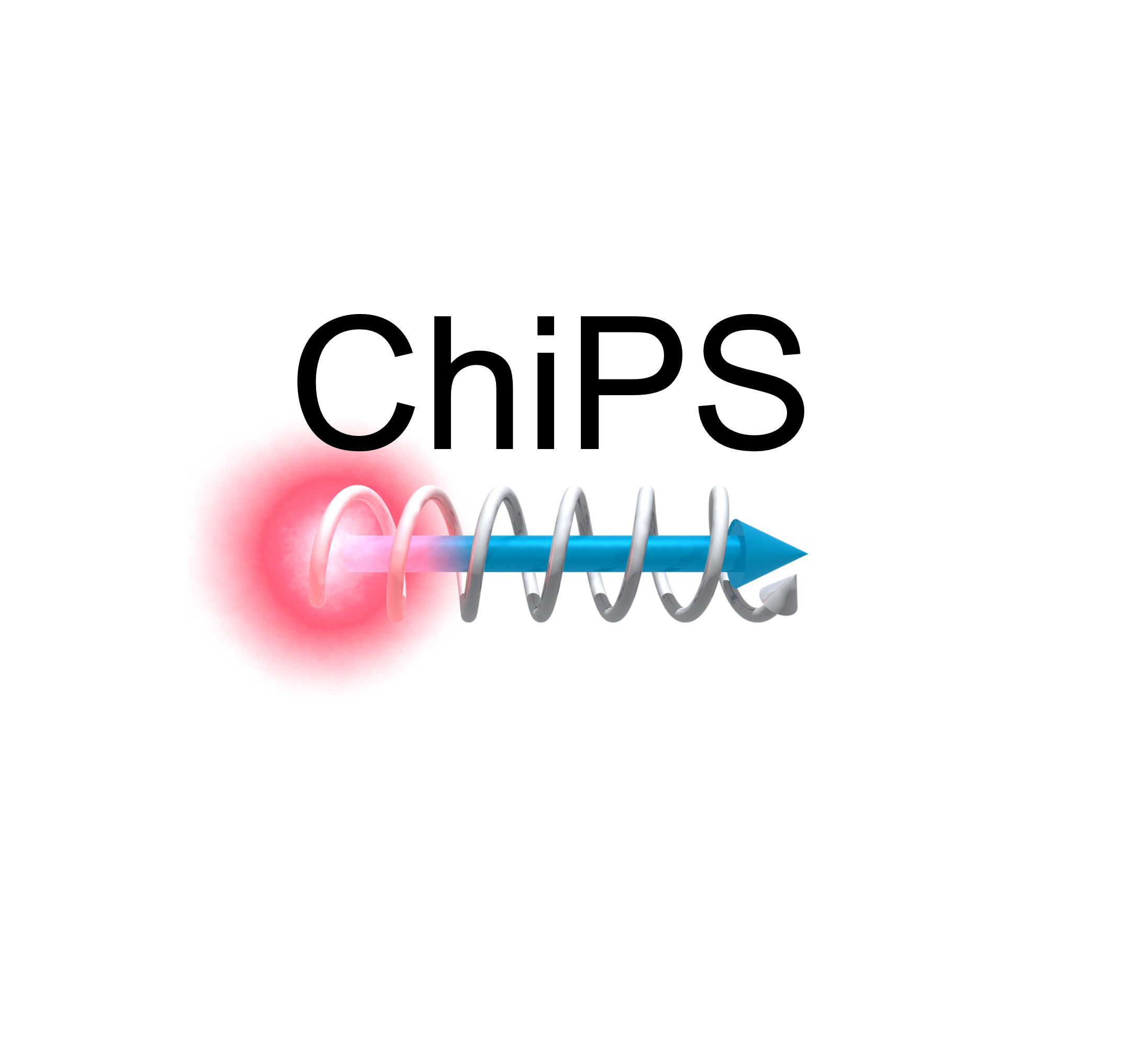
At the Walther-Meißner-Institute, we focus on the investigation of the nature of angular momentum and its transport through lattice excitations, i.e. phonons. The angular momentum in lattice excitations can be described as the rotational motion of the atoms around their rest position, which can be generated by a suitable superposition of linear vibrations or is caused by the symmetry of the lattice. The investigation of angular momentum in phonons requires the ability to excite phonons in a controlled manner with a defined sense of rotation or circular polarization. For this purpose, we will use magnetic excitations with their precession chirality given by the gyromagnetic ratio by coupling a magnetic system to the phononic system. We plan to study the transfer of angular momentum between the magnetic and lattice excitations, to use this to generate phononic angular momentum and its detection, and thus to study angular momentum transport both in bulk and in phononic micro- and nanostructures with tailored phononic properties.
Coordinator: Uli Nowak (University of Konstanz)
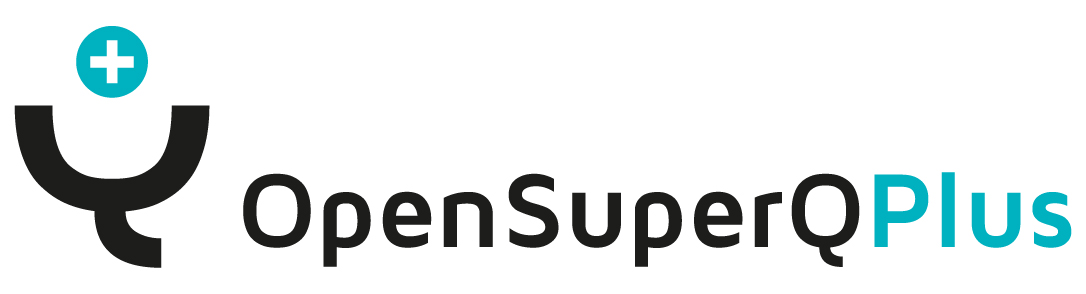
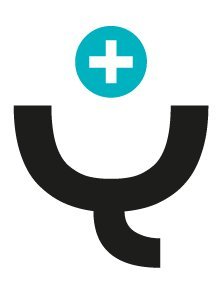
Divided into two 3.5-year project phases, OpenSuperQPlus has an ambitious seven-year agenda with the ultimate goal of a versatile 1,000-qubit quantum-computing system made in Europe. The large-scale consortium anticipates special use cases in quantum simulation for the chemical industry, materials science or in solving optimisation problems and in machine learning.The world is currently taking the first steps into the second quantum revolution where quantum technologies will play a decisive part in advanced technology. In the last few years, quantum computing has been elevated from a basic research concept towards a viable cloud quantum computer which will be affecting people’s everyday lives in multiple ways. The 28 international partners from academia and industry involved in the European FET Flagship project OpenSuperQPlus aim at designing, building, and operating a quantum information processing system of up to 1,000 qubits and to sustainably make it available at a central site for external users.
The scale of the computer will be among the leading platforms in the world and one of the first developed in Europe. To maximise the impact of the project in the field, the OpenSuperQPlus team strives to establish close links with European and international research and industry players as technology partners and users. Highly recognised stakeholders will contribute to the success of OpenSuperQPlus through their participation in the planned advisory board, the basic science group, and a user board.
OpenSuperQPlus will provide access to the machine thus fostering scientific and exploitation progress which does not depend on the investment of individual players. One of the main results will be designing a plan for scaling up and constructing a large-scale quantum computer with at least 1,000 qubits usable for fault-tolerant quantum computing.
About the Quantum Flagship
The Quantum Flagship was launched in 2018 as one of the largest and most ambitious research initiatives of the European Union. With a budget of €1 billion and a duration of 10 years, the flagship brings together research institutions, academia, industry, enterprises, and policy makers, in a joint and collaborative initiative on an unprecedented scale. The main objective of the Flagship is to consolidate and expand European scientific leadership and excellence in this research area as well as to transfer quantum physics research from the lab to the market by means of commercial applications and disruptive technologies. With over 5,000 researchers from academia and industry involved in this initiative throughout its lifetime, it aims to create the next generation of disruptive technologies that will impact Europe’s society, placing the region as a worldwide knowledge-based industry and technological leader in this field.
Frank Wilhelm-Mauch (Forschungszentrum Jülich)
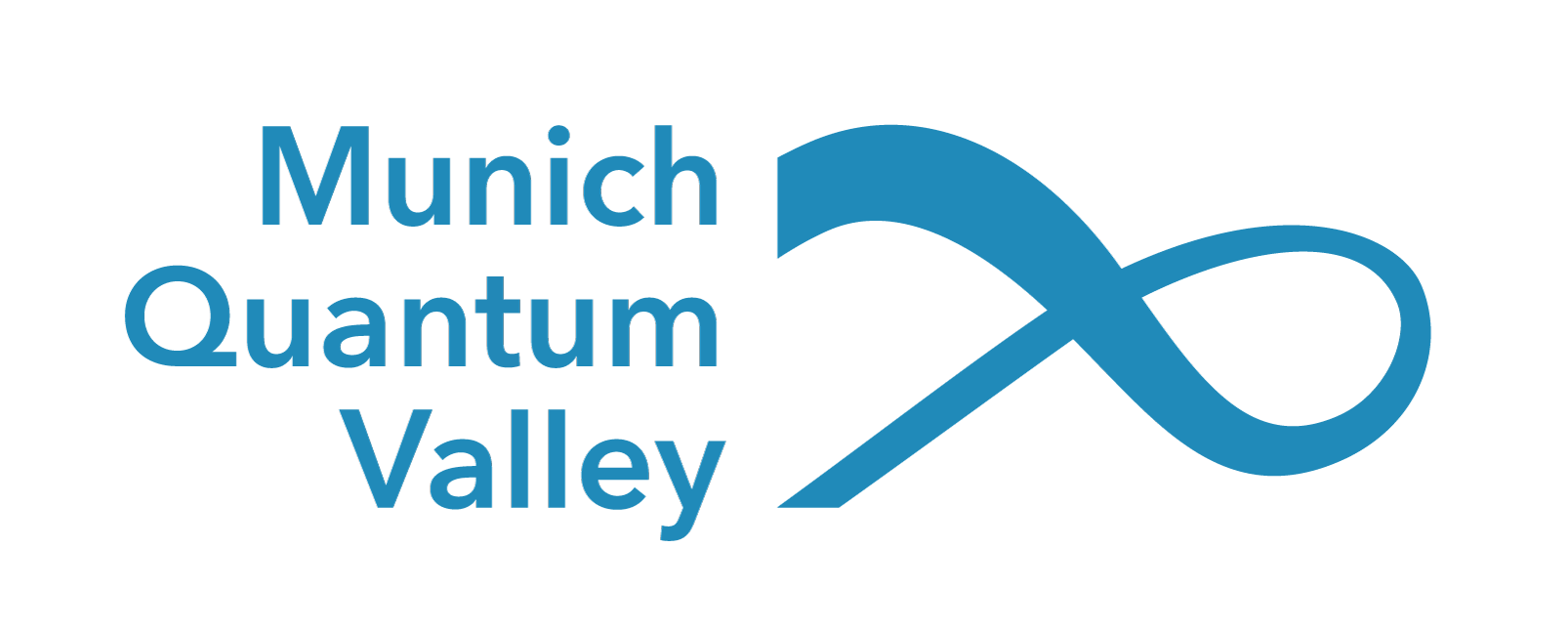
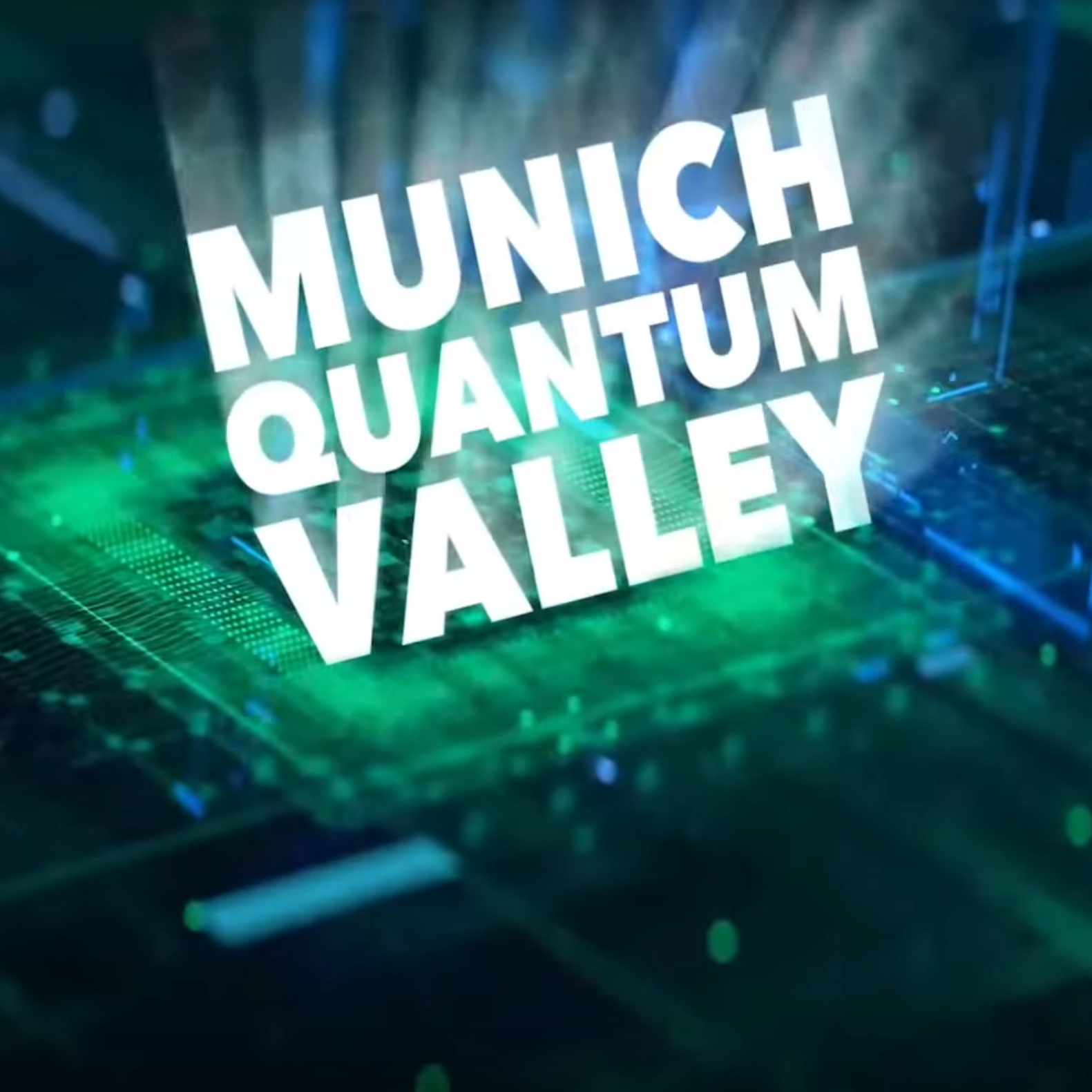
In August 2020, the spokespersons of the Excellence Cluster MCQST – Immanuel Bloch, Ignacio Cirac and Rudolf Gross – started an initiative to establish the Munich Quantum Valley. In a strategy paper, written with support of Max-Planck Vice President Klaus Blaum and FhG Research Director Raoul Klingner, they pointed out that the larger Munich area with its excellent research institutions as well as its active industrial, high-tech and venture capital environment is ideally suited to establish a unique European center for quantum science and technology (QST).
Financial support of MQV was immediately included into the Bavarian «Hightech Agenda Plus». Meanwhile, a Memorandum of Understanding has been signed by Prime Minister Söder and the presidents of the five founding research organizations (see MQV Kick-off event). For 2022/23, a budget of 120 Mio. € is already allocated and a total budget of 300 Mio. € is planned. By MQV, Bavaria as a whole will be developed into a leading international center with the potential to attract the best researchers and open up new opportunities for Bavaria as a business location in this innovative field. To implement MQV, the following three-point plan was proposed in the strategy paper will be implemented:
- Foundation of a Center for Quantum Computing and Quantum Technology to foster networking with industry, to develop priorities in QST R&D, and to coordinate the allocation of funding with the following priorities:
- Development, realization and operation of quantum computers based on a longterm institutional funding to guarantee international competitiveness.
- Support of basic science and development of basic quantum technologies within so-called lighthouse projects.
- Technology transfer to industry and start-ups.
- Establishment of a Quantum Technology Park to provide the technological infrastructure for the development and fabrication of quantum devices.
- Qualification and Education of the next generation of quantum experts in natural and computer sciences as well as engineering, including training and re-education of skilled employees in industry.
Initiated by:
Klaus Blaum, Immanuel Bloch, Ignacio Cirac, Rudolf Gross, Raoul Klingner
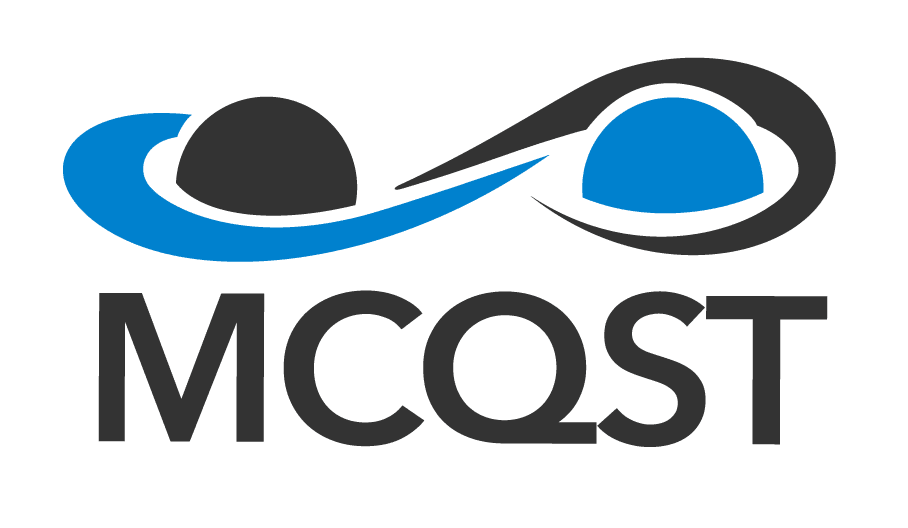
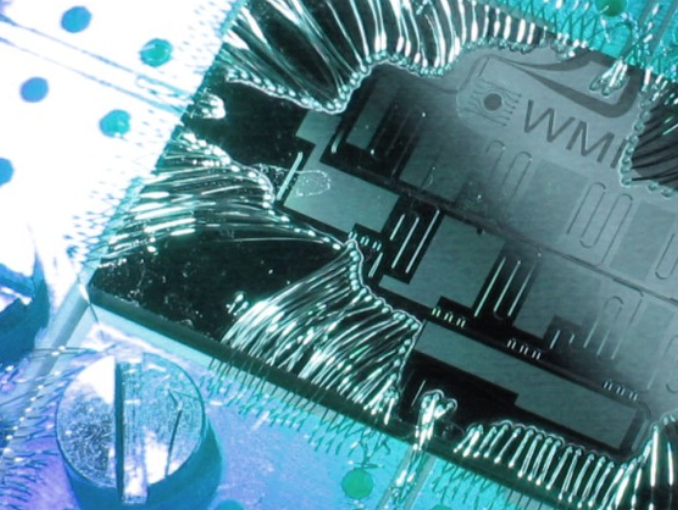
Quantum Mechanics and Information Science have revolutionized our modern world beyond imagination. Whilst quantum mechanics forms the basis for our understanding of the microscopic world, information science is the basic building block for information processing and communication in our digital age. Today we are witnessing a scientific and technological revolution in which Information Science and Quantum Mechanics no longer stand as separate entities, but have rather been united in the common language of Quantum Information Science. First developed to describe the working principles of future Quantum Computers, Quantum Information Science has emerged as an even more powerful description of our physical world, with wide ranging relevance, directly linking fields such as quantum materials and quantum chemistry to seemingly disparate fields such as the cosmology of black holes. At the core of this description is the notion of entanglement, an essential feature without any classical analogue that is responsible for a plethora of astonishing phenomena and applications of Quantum Physics.
These dramatic developments have led to the new combined research field of Quantum Science and Technology (QST), in which these diverse topics, their interconnection as well as their consequences for practical applications are being explored. QST unites multidisciplinary research across physics, mathematics, computer science, electrical engineering, materials science, chemistry, and recently, even cosmology and high energy physics.The core goal of the Munich Center for Quantum Science and Technology (MCQST) is to discover and understand the novel and unifying concepts in the interdisciplinary research fields of QST and to make them tangible and practical, to develop the extraordinary applications within reach by building next-generation quantum devices.
At a fundamental science level, this includes the comprehensive understanding and control of entanglement in quantum many-body systems spanning different time, length and energy scales, through novel theoretical and experimental approaches in quantum information science. Applications for Quantum Devices and Materials to be developed at MCQST range from inherently secure communication and processing of information to ultrasensitive sensors and transducers for precision metrology.Munich is in a unique position to form such a world-leading research center in QST due to its longstanding experience, broad and proven interdisciplinary expertise, and outstanding excellence of the participating senior and junior researchers in all core fields of QST. Developing education and support for junior researchers in QST as well as advancing the strengths of Munich research structures within MCQST will ensure long-term and high-impact research as well as an ideal entry point for industry in this increasingly important field. It will allow Munich to achieve an outstanding visibility and assume a leading position in QST research.
Immanuel Bloch (LMU, MPQ)
Ignacio Cirac (TUM, MPQ)
Rudolf Gross (BAdW, TUM)

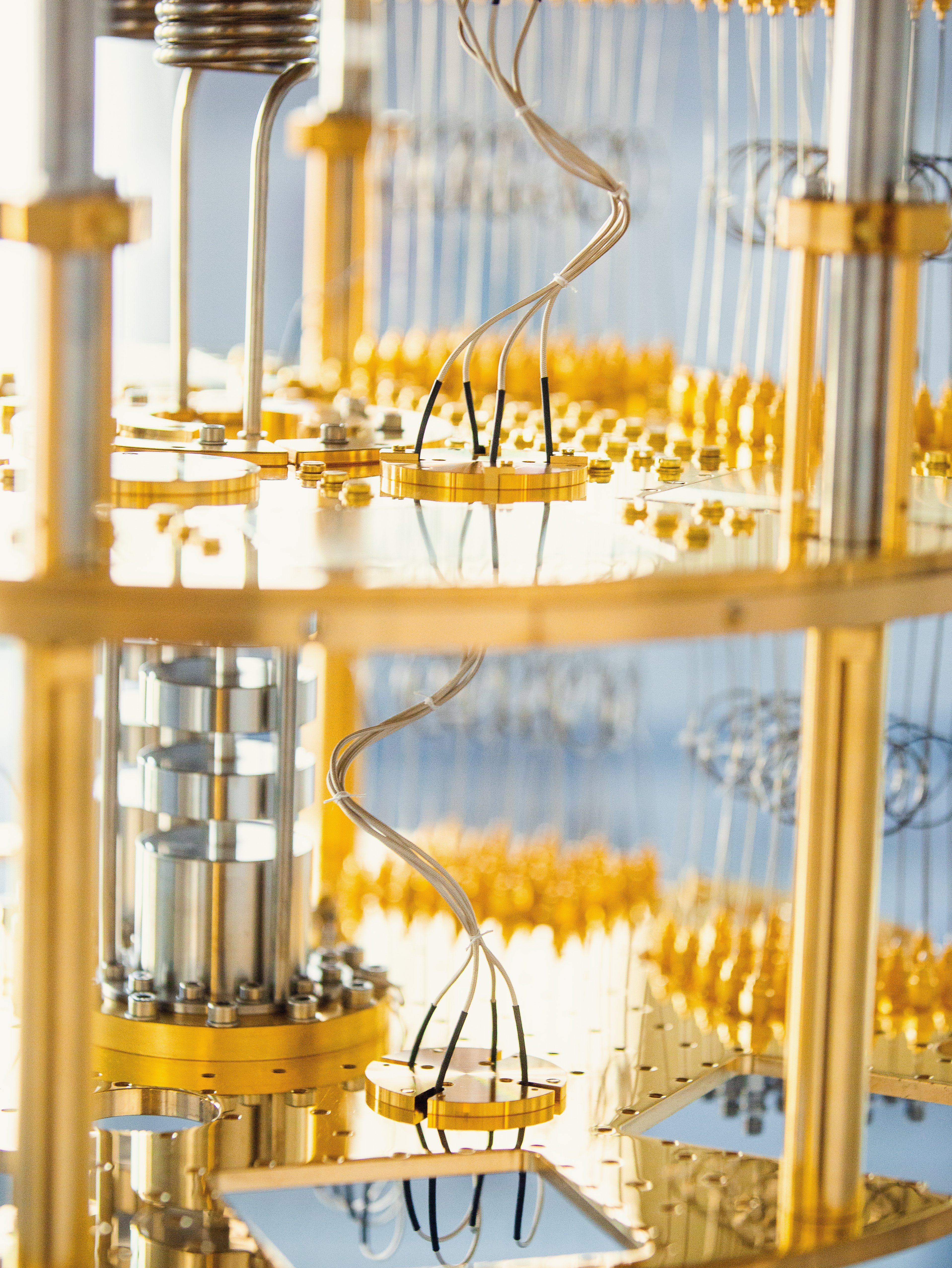
Stefan Filipp (WMI)

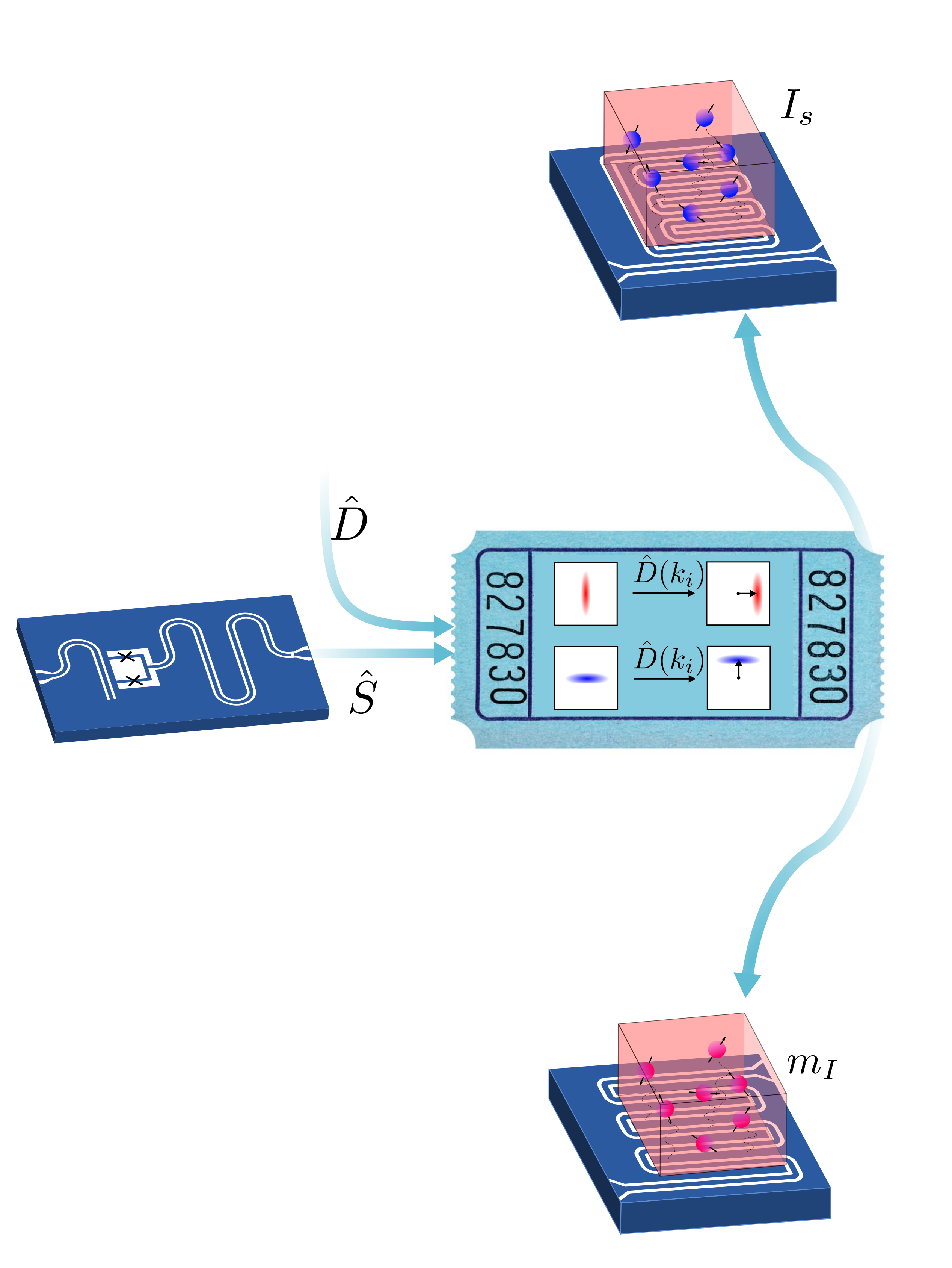
The core objective of this collaborative project is the realization, investigation and demonstration of quantum tokens (Q-tokens) in the microwave or GHz frequency range. The quantum tokens will be implemented in the form of quantum keys stored in quantum memories based on spin ensembles. In general, scalable and long-lived quantum memories represent one of the missing elements needed to build local microwave-based quantum networks. Quantum tokens in the form of propagating squeezed states represent a particularly important use case for such quantum networks.
To achieve our ambitious goals, we identify the following work packages which correspond to individual sub-projects within WMI:
- Experimental realization, characterization and optimization of Q-token generation using time and frequency multiplexing techniques (PI: Kirill Fedorov).
- Experimental realization, characterization and improvement of a rare-earth spin-based microwave quantum memory, including storage and retrieval of generated Q-tokens encoded in frequency domain (PI: Nadezhda Kukharchyk).
- Experimental realization, characterization and improvement of a microwave quantum memory based on phosphorous donors in silicon, including storage and retrieval of generated Q-tokens encoded in time-domain (PI: Hans Hübl).
The generation of Q-tokens in the GHz-frequency range can be achieved by exploiting displaced squeezed states. The latter are generated with the help of superconducting Josephson parametric amplifiers. The actual quantum memories are realized with spin ensembles having transition frequencies in the GHz range. They will be realized by two approaches: the storage of keys in (i) the rare-earth ion system 167Er:Y2SiO5, and (ii) in the isotopically-purified 28Si:P donor system coupled to a microwave resonator. These different spin systems have mutually exclusive advantages, and therefore, both will have useful applications in certain quantum communication scenarios.
Achieving our key goals will require a close coordination between the sub-projects by combining those into final joint experiments towards successful storage of microwave Q-Tokens in quantum spin memories.
Dr. Nadezhda Kukharchyk (WMI)
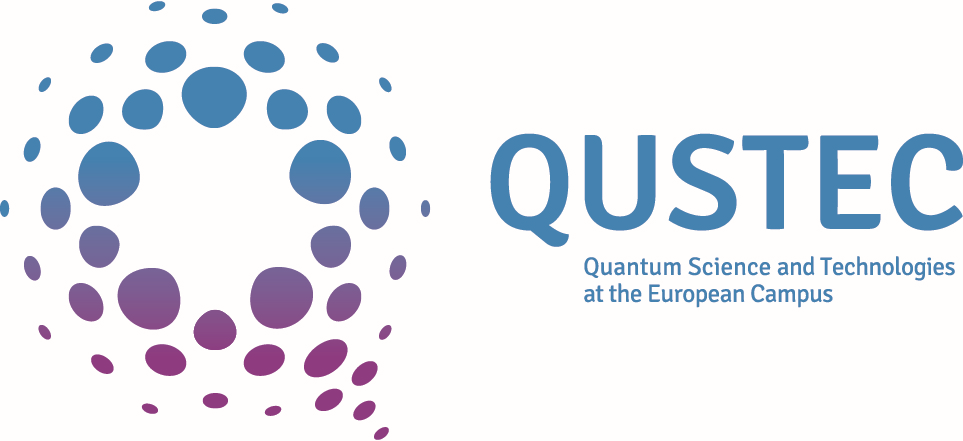
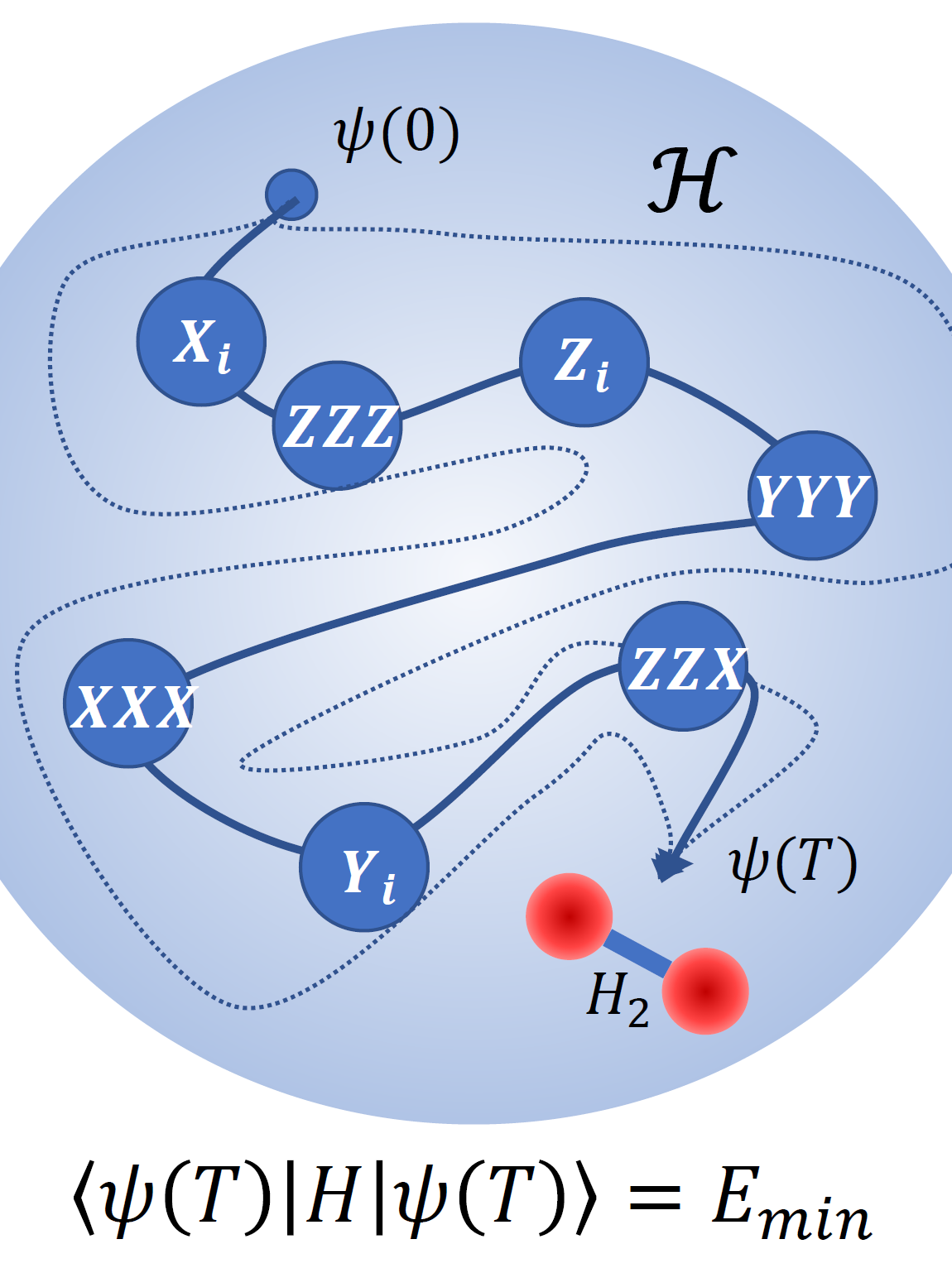
We design and realize parametric couplers connecting multiple superconducting qubits and investigate multi-qubit operations that allow us to entangle multiple qubits at the same time. We explore multi-qubit entangling interactions and evaluate the maximally possible number of qubits coupled to a single coupler. We aim to address the question if there is an advantage in using multi-qubit gates over traditional two-qubit gates in practical experiments. The devices and methods developed in this project will enhance the scalability of superconducting qubit platforms and the efficiency of quantum algorithms.
Prof. Guido Pupillo, Univ. Strasbourg
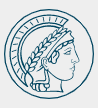
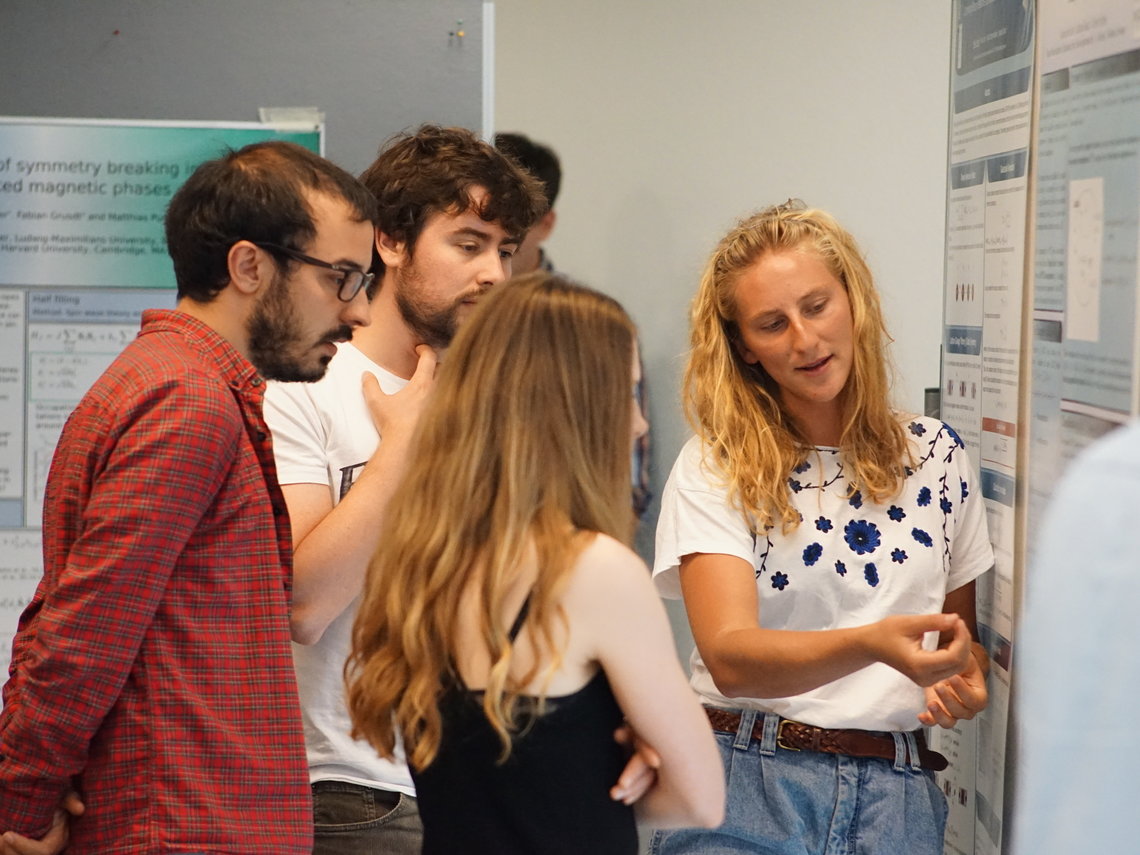
The International Max Planck Research School for Quantum Science and Technology is a joint program of the Max Planck Institute of Quantum Optics, the Ludwig-Maximilians-Universität München and the Technical University of Munich. It offers an excellent and coherent graduate program across the fields of atomic physics, quantum optics, solid state physics, material science, quantum information theory, and quantum many-body systems.
First and foremost, IMPRS-QST provides a platform of joint activities for a large research community, encouraging better networking and scientific exchange as an integral part of doctoral training.
IMPRS-QST students can either get directly admitted to the program through our yearly application process or join as members after starting their PhD at one of our associated research groups.
Ignacio Cirac (MPQ, TUM)
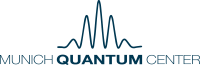
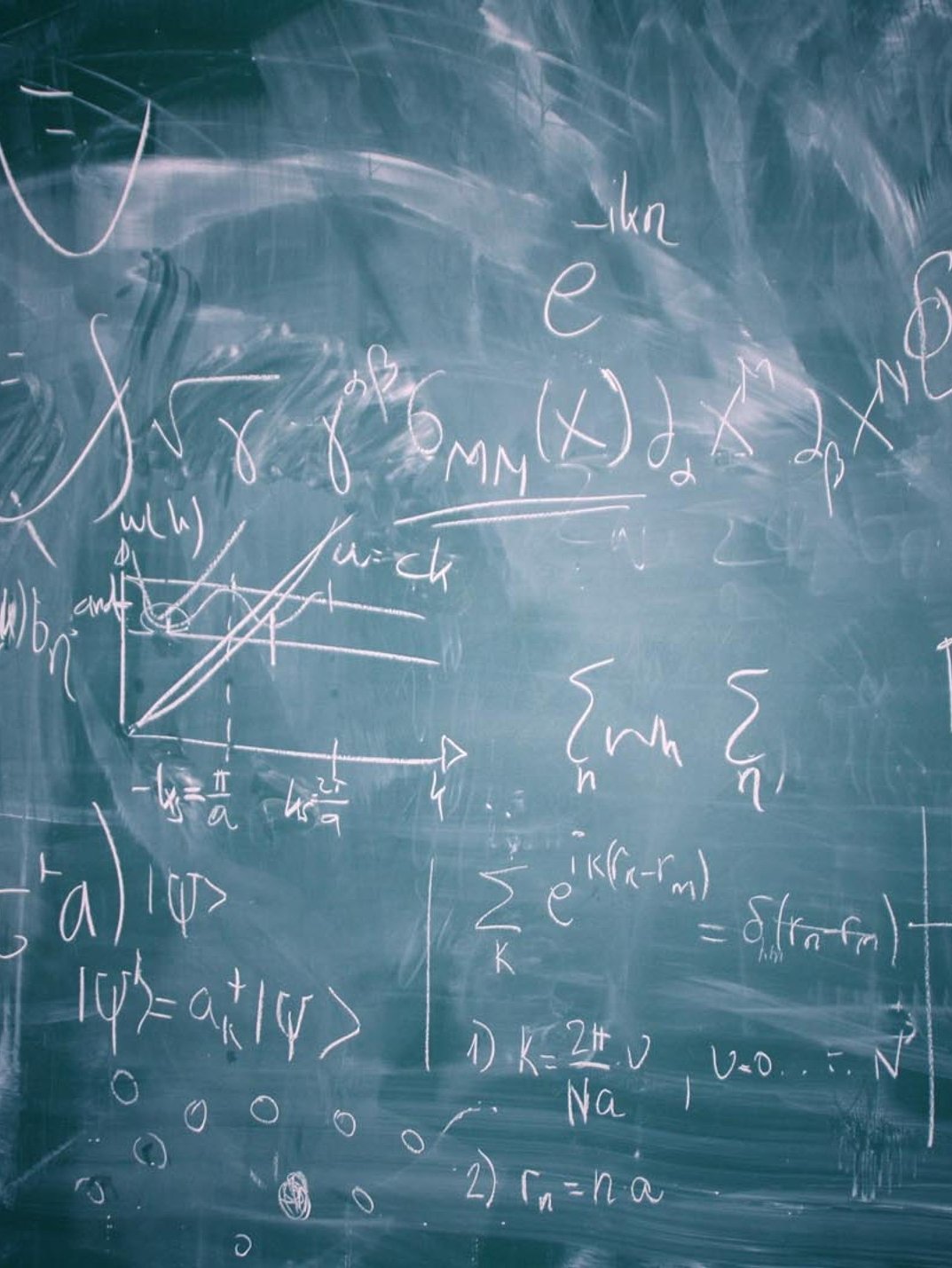
In the greater Munich area there is an extremely active cluster of institutions and research centers committed to the highest standards of excellence in research and teaching in the field of quantum science and technology.
The members and principal investigators of the Munich Quantum Center (MQC) research groups meet up regularly at common workshops and seminars to create a very interactive ambience for quantum science in Munich. The MQC was born at the heart of this vivid atmosphere, gathering over 50 research groups belonging to four different institutions: the Ludwig-Maximilians-Universität München, the Technical University of Munich, the Max Planck Institute of Quantum Optics, and the Walther-Meißner-Institute for Low Temperature Research.
Our research covers a wide array of topics ranging from mathematical foundations, quantum information, computational methods, quantum nano-systems, quantum optics, and quantum many-body physics to superconducting quantum devices.
Tatjana Wilk, LMU München
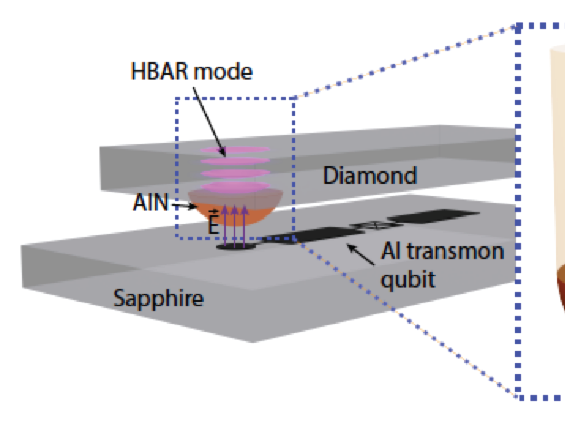
While the experimental implementation of the acoustic interface will be mainly carried out by the groups of Prof. Yiwen Chu and Prof. Christian Degen at ETH Zurich, the Theory Division at WMI, led by Peter Rabl, will be responsible for modelling the system and for developing optimized transfer protocols, which are compatible with the weak spin-phonon couplings and relevant decoherence processes in the system. Here the team can build on the existing expertise in the group on spin-phonon interactions, phononic quantum networks and noise-mitigation strategies.
Yiwen Chu (ETH Zurich)
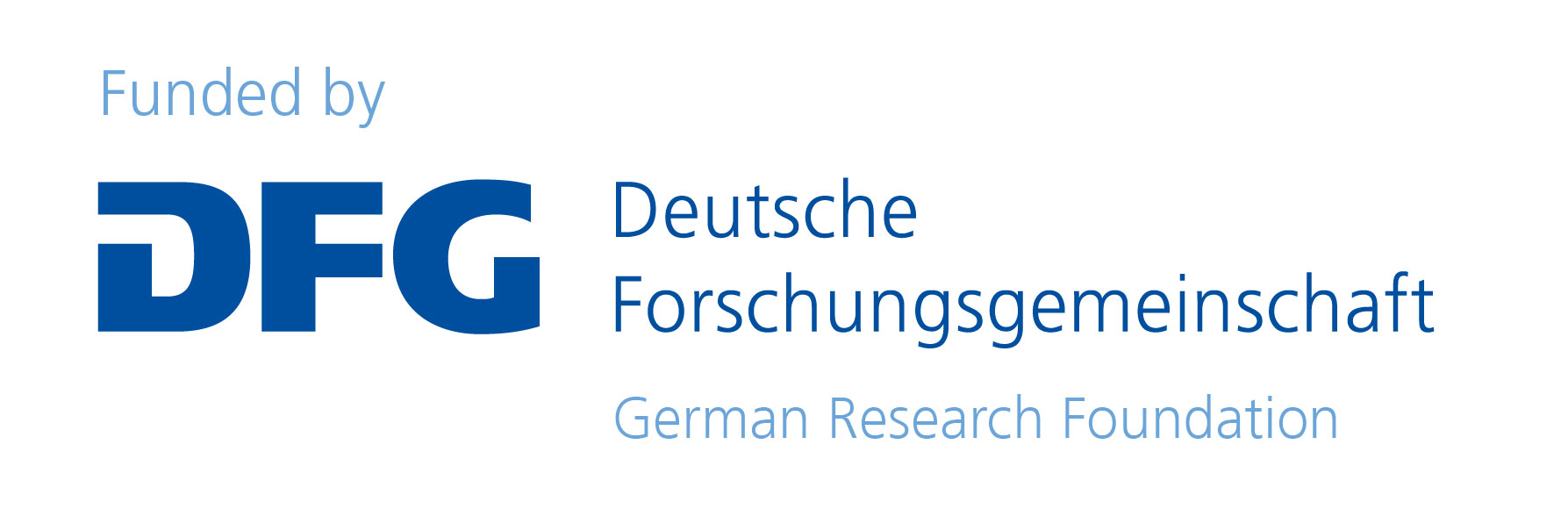
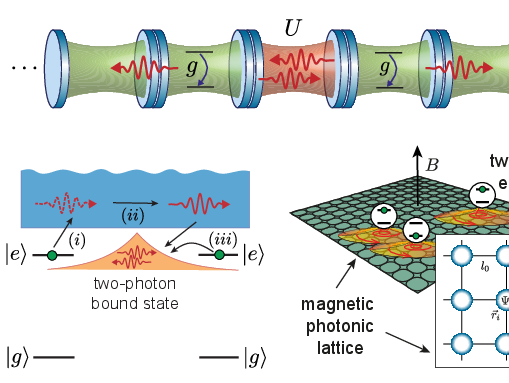
The spontaneous decay of an excited atom is a prototypical example, which shows how the coupling of microscopic quantum systems to a large environment leads to irreversible decay. Such open quantum systems are usually investigated under the premise that the environment can be well-described in terms of a set of independent harmonic oscillators, for example, electromagnetic modes. In this project we will go beyond this assumption and investigate system-reservoir interactions under conditions where excitations in the environment are strongly interacting. This research is primarily motivated by the goal to understand the physics of light-matter interactions in so-called waveguide QED systems, where due to a strong transverse confinement of the field, nonlinear effects at the few-photon level become significant and experimentally observable. The overall goal of this project is, first of all, to systematically investigate and characterize the most fundamental decay and scattering processes that arise in nonlinear photonic waveguides and to identify experimental configurations, where these effects dominate over the usual dissipation dynamics in linear environments. In a second step we will then investigate the application of these effects for new photo-detection and quantum information processing schemes, but also extend our analysis to topological photonic systems in 2D. Here, the interplay between strong light-matter interactions and photon nonlinearities leads to a particularly rich phenomenology and we will show that this can be exploited to implement new control and detection schemes for photonic quantum (Hall) simulators.
Peter Rabl
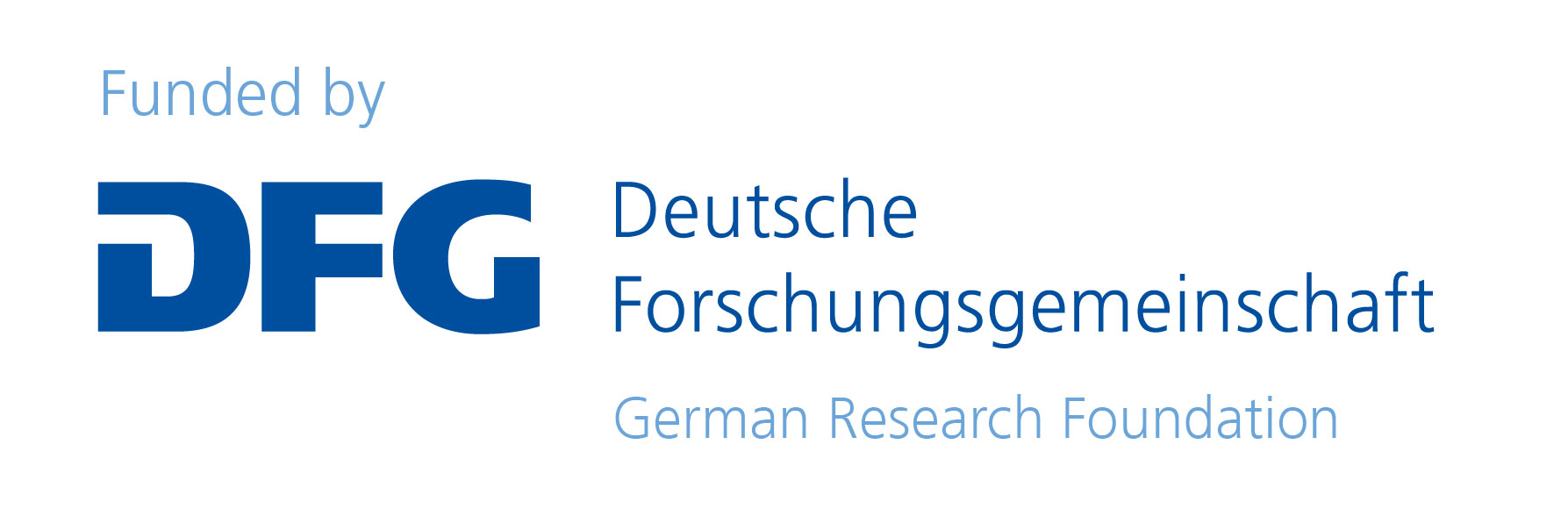
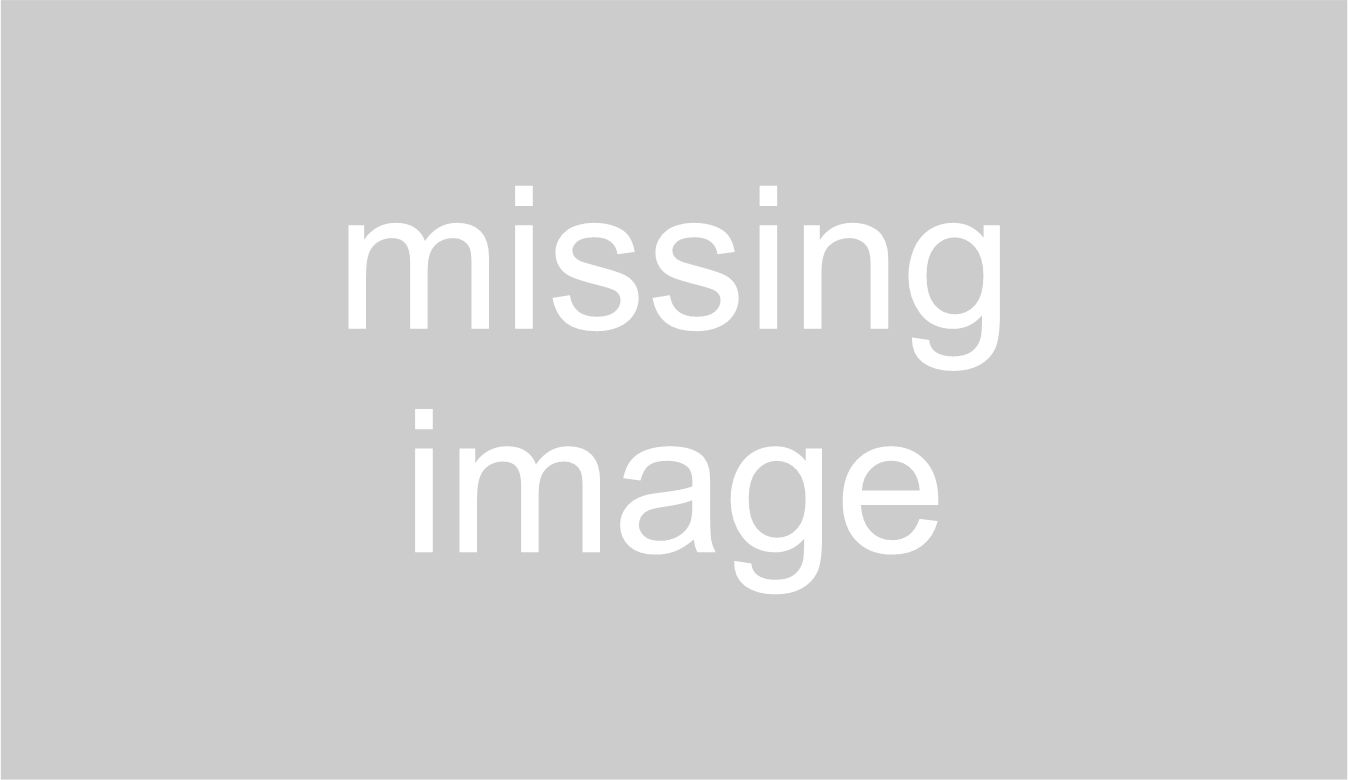
This quantum algorithm determines the groundstate of a given Hamiltonian, for example a molecular electronic configuration Hamiltonian. The quantum state of the system is steered to the target state by varying parameters of a gate sequence on the qubits to optimize a cost function on a classical computer. The advantage of such a hybrid quantum-classical computation over a purely classical one is that high-dimensional multi-qubit states can be stored efficiently on the quantum device, which is not possible on a classical memory because of the exponentially large number of state coefficients. The challenge on today’s quantum computers is, however, that the VQE algorithm has to converge to the target state before decoherence sets in. It's circuit-depth must be short. The main aim of this project is, therefore, to explore the efficient generation of multi-qubit states going beyond the current paradigm of decomposing all state manipulations into single and two-qubit gates. We will investigate multi-qubit operations that will allow us to entangle multiple qubits at the same time. This will result in short-depth efficient algorithms provided that the fidelity of the multi-qubit gate can be kept high. We use fixed-frequency transmon qubits and two-qubit gates based on parametrically driven tunable couplers. We address the question if there is an advantage in using multi-qubit gates over traditional two-qubit gates not only in theory but also in practical experiments. While theoretically the answer is likely to be affirmative, on the experimental side it is not clear what gate fidelities can be reached and how these compare to a decomposition of multi-qubit gates into two-qubit interactions. We explore N-way tunable couplers that are either capacitively and galvanically coupled to N qubits and evaluate the maximum number of qubits. We investigate multi-qubit entangling interactions via parametric frequency-modulation of the coupler. Different methods are compared, such as resonant or dispersive interactions based on simultaneous pulses to generate different classes of entangled states. The final goal is a four-qubit experiment targeting a quantum chemistry problem, such as determining the ground state and energy spectrum of molecular hydrogen (H2), and to assess the efficiency of multi-qubit gates. It is straightforward to then extend the methods that are tested in this project with a few qubits to larger systems to bring practical applications closer within reach by adding building blocks with higher connectivity and multi-qubit gate capabilities.
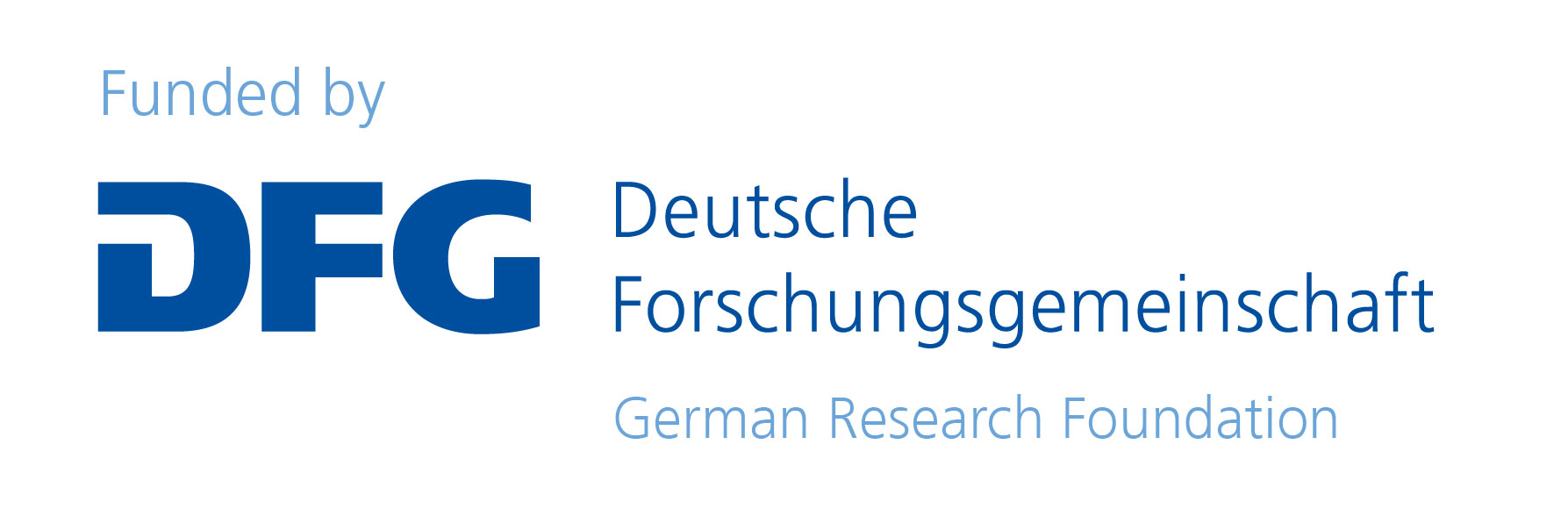
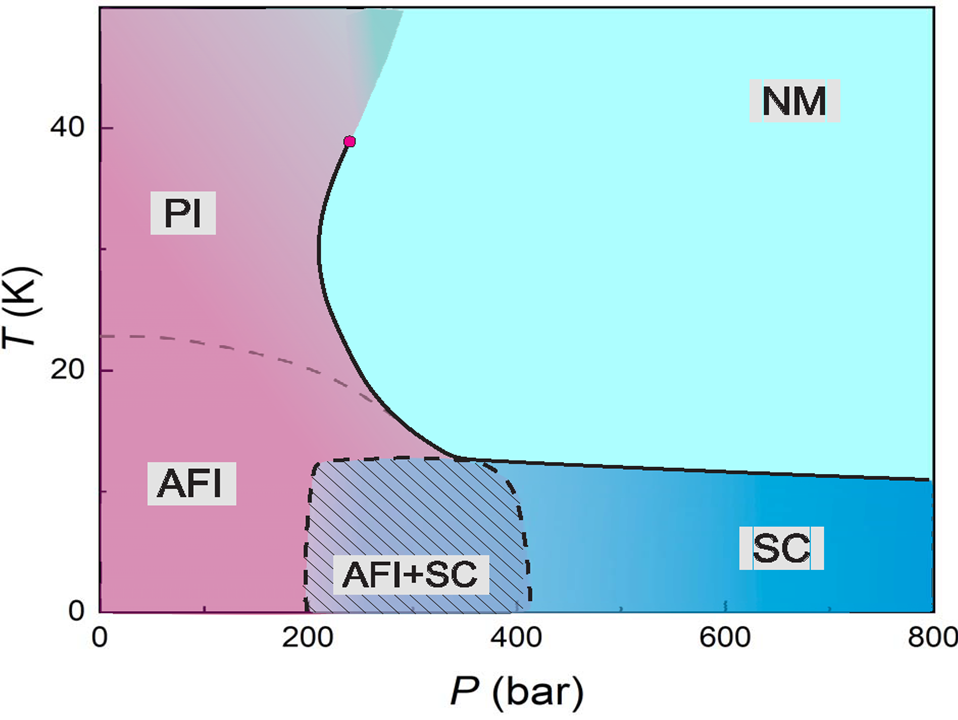
Within this project, we join experts from experimental and theoretical physics and materials science to tackle these problems. We will employ kappa-type organic charge transfer salts as quasi-2D electronic model systems with bandwidth-controlled Mott instability for tracking the evolution of key characteristics of the charge carriers in the metal/insulator coexistence region of the phase diagram as well as in the neighboring homogeneous metallic state. In particular, we will study (i) the correlation-induced renormalization of the effective mass, (ii) the exact geometry and topology of the Fermi surface, and (iii) the coherence of charge transport. The systematic study of these characteristics in well-defined model systems will provide a crucial test for the existing theories of the Mott metal-insulator transition.A key objective of the project is the disentanglement of contributions of charge, spin, and lattice degrees of freedom to the metal-insulator instability. This problem will be addressed by studying kappa-type salts with different strength of geometrical frustration, magnetic interactions, and lattice disorder. The salts of BEDT-TTF with different anions are particularly suited for studying the effects of geometrical frustration, while BETS salts with localized magnetic moments give access to the interplay between electronic correlations and magnetic interactions. The coupling of the electronic state to lattice degrees of freedom will be probed by studying the influence of deuteration of BEDT-TTF salts on the charge carrier properties and by tracing the impact of ethylene-group disorder in these salts.The main experimental probes for addressing the above issues will be magnetic quantum oscillations, semiclassical anisotropic magnetotransport, and resistivity anisotropy. A precise control of the electronic ground state with respect to the metal-insulator boundary will be realized by fine-tuning materials with quasi-hydrostatic pressure as well as "chemical pressure". A quantitative analysis of the experimental results will be carried out by the theory team involved in the project using the state-of-the-art theory of high-field magnetotransport in quasi-2D metals as well as of the charge transport in phase-separated electronic media. Further development of the (magneto)transport theory in the segments related to the proposed experiments is planned.The availability of top-quality single crystals of kappa-type salts is crucial for the success of the planned project. The preparation and characterization of such crystals will be carried out by the experienced materials science team of the project.